Amino Acids
The
Amino Acids
Proteins are formed by polymerizing
monomers that are known as amino acids
because they contain an amine (-NH2) and a
carboxylic acid (-CO2H) functional group. With the
exception of the amino acid proline, which is a secondary
amine, the amino acids used to synthesize proteins are
primary amines with the following generic formula.
 |
An amino acid |
These compounds are known as a-amino acids because the -NH2
group is on the carbon atom next to the -CO2H
group, the so-called carbon atom of the carboxylic acid.

Zwitterions
The chemistry of amino acids is complicated by the fact
that the -NH2 group is a base and the -CO2H
group is an acid. In aqueous solution, an H+ ion
is therefore transferred from one end of the molecule to the
other to form a zwitterion (from the German
meaning mongrel ion, or hybrid ion).
Zwitterions are simultaneously electrically charged and
electrically neutral. They contain positive and negative
charges, but the net charge on the molecule is zero.

The Amino Acids
Used to Synthesize Proteins
More than 300 amino acids are listed in the Practical
Handbook of Biochemistry and Molecular Biology, but only
the twenty amino acids in the table below are used to
synthesize proteins. Most of these amino acids differ only in
the nature of the R substituent. The standard amino
acids are therefore classified on the basis of these R
groups. Amino acids with nonpolar substituents are said to be
hydrophobic (water-hating). Amino acids with polar R
groups that form hydrogen bonds to water are classified as hydrophilic
(water-loving). The remaining amino acids have substituents
that carry either negative or positive charges in aqueous
solution at neutral pH and are therefore strongly
hydrophilic.
The 20 Standard Amino Acids
NAME |
STRUCTURE
(AT NEUTRAL pH) |
Nonpolar (Hydrophobic) R
Groups |
Glycine (Gly) |
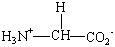 |
Alanine (Ala) |
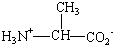 |
Valine (Val) |
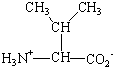 |
Leucine (Leu) |
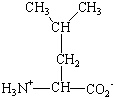 |
Isoleucine (Ile) |
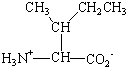 |
Proline (Pro) |
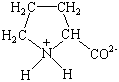 |
Methionine (Met) |
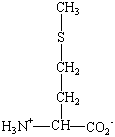 |
Phenylalanine (Phe) |
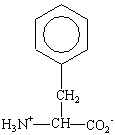 |
Tryptophan (Trp) |
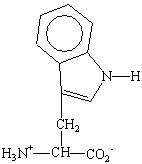 |
Polar (Hydrophilic) R
Groups |
Serine
(ser) |
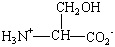 |
Threonine
(Thr) |
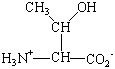 |
Tyrosine
(Tyr) |
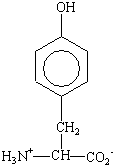 |
Cysteine
(Cys) |
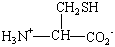 |
Asparagine
(Asn) |
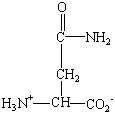 |
Glutamine
(Gln) |
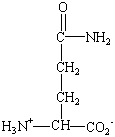 |
Negatively Charged R
Groups |
Aspartic acid (Asp) |
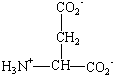 |
Glutamic acid
(Glu) |
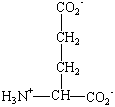 |
Positively Charged R
Groups |
Lysine
(Lys) |
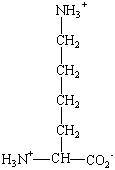 |
Arginine
(Arg) |
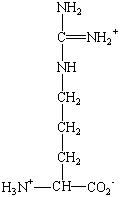 |
Histidine
(His) |
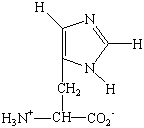 |
|

Amino Acids as Stereoisomers
With the exception of glycine, the common amino acids all
contain at least one chiral carbon atom. These amino acids
therefore exist as pairs of stereoisomers. The structures of
the D and L isomers of alanine are shown in the figure below.
Although D amino acids can be found in nature, only the L
isomers are used to form proteins. The D isomers are most
often found attached to the cell walls of bacteria and in
antibiotics that attack bacteria. The presence of these D
isomers protects the bacteria from enzymes the host organism
uses to protect itself from bacterial infection by
hydrolyzing the proteins in the bacterial cell wall.
 |
|
 |
D-Alanine |
|
L-Alanine |
A few biologically important derivatives of
the standard amino acids are shown in the figure below.
Anyone who has used an "anti-histamine" to
alleviate the symptoms of exposure to an allergen can
appreciate the role that histamine
a
decarboxylated derivative of histidine
plays
in mediating the body's response to allergic reactions.
L-DOPA, which is a derivative of tyrosine, has been used to
treat Parkinson's disease. This compound received notoriety a
few years ago in the film Awakening, which
documented it's use as a treatment for other neurological
disorders. Thyroxine, which is an iodinated ether of
tyrosine, is a hormone that acts on the thyroid gland to
stimulate the rate of metabolism.
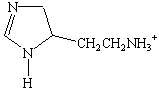 |
Histamine |
|
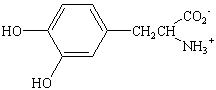 |
L-DOPA |
|
|
|
|
|
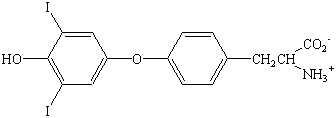 |
|
Thyroxine |
|

The Acid-Base
Chemistry of the Amino Acids
Acetic acid and ammonia often play an important role in
the discussion of the chemistry of acids and bases. One of
these compounds is a weak acid; the other is a weak base.
Thus, it is not surprising that an H+ ion is
transferred from one end of the molecule to the other when an
amino acid dissolves in water.
The zwitterion is the dominant species in aqueous
solutions at physiological pH (pH
7). The
zwitterion can undergo acid-base reactions, howeer, if we add
either a strong acid or a strong base to the solution.
Imagine what would happen if we add a strong acid to a
neutral solution of an amino acid in water. In the presence
of a strong acid, the -CO2- end of this
molecule picks up an H+ ion to form a molecule
with a net positive charge.
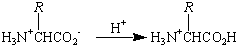
In the presence of a strong base, the -NH3+
end of the molecule loses an H+ ion to form a
molecule with a net negative charge.
The figure below shows what happens to
the pH of an acidic solution of glycine when this amino acid
is titrated with a strong base, such as NaOH.
In order to understand this titration curve, let's start
with the equation that describes the acid-dissociation
equilibrium constant expression for an acid, HA.
Let's now rearrange the Ka expression,
take the log to the base 10 of both sides of
this equation,
and then multiply both sides of the equation by -1.
By definition, the term on the left side of this equation
is the pH of the solution and the first term on the right
side is the pKa of the acid.
The negative sign on this right side of this equation is
often viewed as "inconvenient." The derivation
therefore continues by taking advantage of the following
feature of logarithmic mathematics
to give the following form of this equation.
This equation is known as the Henderson-Hasselbach
equation, and it can be used to calculate the pH of
the solution at any point in the titration
curve.
The following occurs as we go from left to right across
this titration curve.
- The pH initially increases as we add base to the
solution because the base deprotonates some of the
positively charged H3N+CH2CO2H
ions that were present in the strongly acidic
solution.
- The pH then levels off because we form a buffer
solution in which we have reasonable concentrations
of both an acid, H3N+CH2CO2H,
and its conjugate base, H3N+CH2CO2-.
- When virtually all of the H3N+CH2CO2H
molecules have been deprotonated, we no longer have a
buffer solution and the pH rises rapidly when more
NaOH is added to the solution.
- The pH then levels off as some of the neutral H3N+CH2CO2-
molecules lose protons to form negatively charged H2NCH2CO2-
ions. When these ions are formed, we once again get a
buffer solution in which the pH remains relatively
constant until essentially all of the H3N+CH2CO2H
molecules have been converted into H2NCH2CO2-
ions.
- At this point, the pH rises rapidly until it reaches
the value observed for a strong base.
The pH titration curve tells us the volume of base
required to titrate the positively charged H3N+CH2CO2H
molecule to the H3N+CH2CO2-
zwitterion. If we only add half as much base, only half of
the positive ions would be titrated to zwitterions. In other
words, the concentration of the H3N+CH2CO2H
and H3N+CH2CO2-
ions would be the same. Or, using the symbolism in the
Henderson-Hasselbach equation:
[HA] = [A-]
Because the concentrations of these ions is the same, the
logarithm of the ratio of their concentrations is zero.
Thus, at this particular point in the titration curve, the
Henderson-Hasselbach equation gives the following equality.
pH = pKa
We can therefore determine the pKa of
an acid by measuring the pH of a solution in which the acid
has been half-titrated.
Because there are two titratable groups in glycine, we get
two points at which the amino acid is half-titrated. The
first occurs when half of the positive H3N+CH2CO2H
molecules have been converted to neutral H3N+CH2CO2-
ions. The second occurs when half of the H3N+CH2CO2-
zwitterions have been converted to negatively charged H2NCH2CO2-
ions.
The following results are obtained when this technique is
applied to glycine.
Let's compare these values with the pKa's of
acetic acid and the ammonium ion.
CH3CO2H |
|
|
pKa = 4.74 |
|
NH4+ |
|
|
pKa = 9.24 |
|
The acid/base properties of the a-amino group in an amino acid are very
similar to the properties of ammonia and the ammonium ion.
The a-amine, however, has a
significant effect on the acidity of the carboxylic acid. The
-amine increases the value of Ka for the
carboxylic acid by a factor of about 100.
The inductive effect of the a-amine
can only be felt at the a-CO2H
group. If we look at the chemistry of glutamic acid, for
example, the a-CO2H
group on the R substituent has an acidity that is
close to that of acetic acid.
When we titrate an amino acid from the low end of the pH
scale (pH
1) to the high end (pH
13), we
start with an ion that has a net positive charge and end up
with an ion that has a net negative charge.
Somewhere between these extremes, we have to find a
situation in which the vast majority of the amino acids are
present as the zwitterion
with no net electric charge. This point is called the isoelectric
point (pI) of the amino acid.
For simple amino acids, in which the R group
doesn't contain any titratable groups, the isoelectric point
can be calculated by averaging the pKa
values for the a-carboxylic acid
and a-amino groups. Glycine, for
example, has a pI of about 6.
pI = 2.35 + 9.78 = 6.1
2
At pH
6, more than 99.98% of the glycine molecules in
this solution are present as the neutral H3N+CH2CO2H
zwitterion.
When calculating the pI of an amino acid that has
a titratable group on the R side chain, it is useful
to start by writing the structure of the amino acid at
physiological pH (pH
7). Lysine, for example, could be
represented by the following diagram.
At physiological pH, lysine has a net positive charge.
Thus, we have to increase the pH of the solution to remove
positive charge in order to reach the isoelectric point. The
pI for lysine is simply the average of the pKa's
of the two -NH3+ groups.
pI = 9.18 + 10.79
10.0
2
At this pH, all of the carboxylic acid groups are present
as -CO2- ions and the total population
of the -NH3+ groups is equal to one.
Thus, the net charge on the molecule at this pH is zero.
If we apply the same technique to the pKa
data for glutamic acid, given above, we get a pI of
about 3.1. The three amino acids in this section therefore
have very different pI values.
Glutamic acid |
|
(R = -CH2CH2CO2-):
|
|
pI = 3.1 |
Glycine |
|
(R = -H): |
|
pI = 6.1 |
Lysine |
|
(R = -CH2CH2CH2CH2NH3+): |
|
pI = 10.0 |
Thus, it isn't surprising that a common
technique for separating amino acids (or the proteins they
form) involves placing a mixture in the center of a gel and
then applying a strong voltage across this gel. This
technique, which is known as gel electrophoresis,
is based on the fact that amino acids or proteins that carry
a net positive charge at the pH at which the separation is
done will move toward the negative electrode, whereas those
with a net negative charge will move toward the positive
electrode.

