The Chemistry of
Hemoglobin and Myoglobin
At one time or another, everyone has experienced the
momentary sensation of having to stop, to "catch one's
breath," until enough O2 can be absorbed by
the lungs and transported through the blood stream. Imagine
what life would be like if we had to rely only on our lungs
and the water in our blood to transport oxygen through our
bodies.
O2 is only marginally soluble (< 0.0001 M)
in blood plasma at physiological pH. If we had to rely on the
oxygen that dissolved in blood as our source of oxygen, we
would get roughly 1% of the oxygen to which we are
accustomed. (Consider what life would be like if the amount
of oxygen you received was equivalent to only one breath
every 5 min, instead of one breath every 3 s.) The evolution
of forms of life even as complex as an earthworm required the
development of a mechanism to actively transport oxygen
through the system. Our blood stream contains about 150 g/L
of the protein known as hemoglobin (Hb),
which is so effective as an oxygen-carrier that the
concentration of O2 in the blood stream reaches
0.01 M
the same
concentration as air. Once the Hb-O2 complex
reaches the tissue that consumes oxygen, the O2
molecules are transferred to another protein
myoglobin (Mb)
which transports oxygen through the muscle tissue.
The site at which oxygen binds to both hemoglobin and
myoglobin is the heme shown in the figure
below.
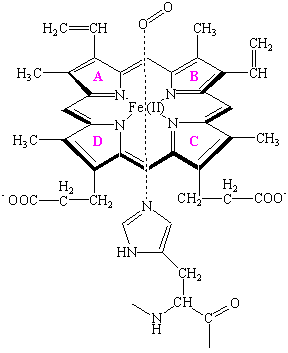
At the center of the heme is an Fe(II) atom. Four of the
six coordination sites around this atom are occupied by
nitrogen atoms from a planar porphyrin ring.
The fifth coordination site is occupied by a nitrogen atom
from a histidine side chain on one of the amino acids in the
protein. The last coordination site is available to bind an O2
molecule. The heme is therefore the oxygen-carrying portion
of the hemoglobin and myoglobin molecules. This raises the
question: What is the function of the globular protein or
"globin" portion of these molecules?
The structure of myoglobin suggests that the
oxygen-carrying heme group is buried inside the protein
portion of this molecule, which keeps pairs of hemes group
from coming too close together. This is important, because
these proteins need to bind O2 reversibly and the
Fe(II) heme, by itself, cannot do this. When there is no
globin to protect the heme, it reacts with oxygen to form an
oxidized Fe(III) atom instead of an Fe(II)-O2
complex.
Hemoglobin consists of four protein chains, each about the
size of a myoglobin molecule, which fold to give a structure
that looks very similar to myoglobin. Thus, hemoglobin has
four separate heme groups that can bind a molecule of O2.
Even though the distance between the iron atoms of adjacent
hemes in hemoglobin is very large
between 250 and 370 nm
the act of
binding an O2 molecule at one of the four hemes in
hemoglobin leads to a significant increase in the affinity
for O2 binding at the other hemes.
This cooperative interaction between
different binding sites makes hemoglobin an unusually good
oxygen-transport protein because it enables the molecule to
pick up as much oxygen as possible once the partial pressure
of this gas reaches a particular threshold level, and then
give off as much oxygen as possible when the partial pressure
of O2 drops significantly below this threshold
level. The hemes are much too far apart to interact directly.
But, changes that occur in the structure of the globin that
surrounds a heme when it picks up an O2 molecule
are mechanically transmitted to the other globins in this
protein. These changes carry the signal that facilitates the
gain or loss of an O2 molecule by the other hemes.
Drawings of the structures of proteins often convey the
impression of a fixed, rigid structure, in which the
side-chains of individual amino acid residues are locked into
position. Nothing could be further from the truth. The
changes that occur in the structure of hemoglobin when oxygen
binds to the hemes are so large that crystals of deoxygenated
hemoglobin shatter when exposed to oxygen. Further evidence
for the flexibility of proteins can be obtained by noting
that there is no path in the crystal structures of myoglobin
and hemoglobin along which an O2 molecule can
travel to reach the heme group. The fact that these proteins
reversibly bind oxygen suggests that they must undergo simple
changes in their conformation
changes
that have been called breathing motions.
that open up and then close down the pathway along which an O2
molecule travels as it enters the protein. Computer
simulations of the motion within proteins suggests that the
interior of a protein has a significant "fluidity,"
with groups moving within the protein by as much as 20 nm.

