The Carbonyl Group
Description
of the Carbonyl Group
It is somewhat misleading to write the carbonyl group as a
covalent C=O double bond. The difference between the
electronegativities of carbon and oxygen is large enough to
make the C=O bond moderately polar. As a result, the carbonyl
group is best described as a hybrid of the following
resonance structures.
We can represent the polar nature of this hybrid by
indicating the presence of a slight negative charge on the
oxygen (d-) and a
slight positive charge (d+)
on the carbon of the C=O double bond.
Reactions at
the Carbonyl Group
Reagents that attack the electron-rich d - end of the C=O bond are
called electrophiles (literally,
"lovers of electrons"). Electrophiles include ions
(such as H+ and Fe3+) and neutral
molecules (such as AlCl3 and BF3) that
are Lewis acids, or electron-pair acceptors.
Reagents that attack the electron-poor d+
end of this bond are nucleophiles
(literally, "lovers of nuclei"). Nucleophiles are Lewis
bases (such as NH3 or the OH-
ion).
The polarity of the C=O double bond can be used to explain
the reactions of carbonyl compounds. Aldehydes and ketones
react with a source of the hydride (H-) ion
because the H- ion is a Lewis base, or
nucleophile, that attacks the d+
end of the C=O bond. When this happens, the two valence
electrons on the H- ion form a covalent bond to
the carbon atom. Since carbon is tetravalent, one pair of
electrons in the C=O bond is displaced onto the oxygen to
form an intermediate with a negative charge on the oxygen
atom.
This alkoxide ion can then remove an H+ ion
from water to form an alcohol.
Common sources of the H- ion include lithium
aluminum hydride (LiAlH4) and sodium borohydride
(NaBH4). Both compounds are ionic.
LiAlH4: |
|
|
[Li+][AlH4-] |
|
|
|
|
NaBH4: |
|
|
[Na+][BH4-] |
The aluminum hydride (AlH4-)
and borohydride (BH4-) ions act as if
they were complexes between an H- ion, acting as a
Lewis base, and neutral AlH3 or BH3
molecules, acting as a Lewis acid.
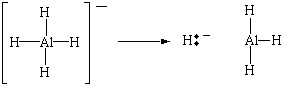
LiAlH4 is such as good source of the H-
ion that it reacts with the H+ ions in water or
other protic solvents to form H2 gas. The first
step in the reduction of a carbonyl with LiAlH4 is
therefore carried out using an ether as the solvent. The
product of the hydride reduction reaction is then allowed to
react with water in a second step to form the corresponding
alcohol.
NaBH4 is less reactive toward protic solvents,
which means that borohydride reductions are usually done in a
single step, using an alcohol as the solvent.

Carboxylic Acids
and Carboxylate Ions
When one of the substituents on a carbonyl group is an OH
group, the compound is a carboxylic acid
with the generic formula RCO2H. These compounds
are acids, as the name suggests, which form carboxylate
ions (RCO2-) by the loss of an
H+ ion.
The carboxylate ion formed in this reaction is a hybrid of
two resonance structures.
Resonance delocalizes the negative charge in
the carboxylate ion, which makes this ion more stable than
the alkoxide ion formed when an alcohol loses an H+
ion. By increasing the stability of the conjugate base,
resonance increases the acidity of the acid that forms this
base. Carboxylic acids are therefore much stronger acids than
the analogous alcohols. The value of Ka
for a typical carboxylic acid is about 10-5,
whereas alcohols have values of Ka
of only 10-16.
Carboxylic acids were among the first organic compounds to
be discovered. As a result, they have well-established common
names that are often derived from the Latin stems of their
sources in nature. Formic acid (Latin formica,
"an ant") and acetic acid (Latin acetum,
"vinegar") were first obtained by distilling ants
and vinegar, respectively. Butyric acid (Latin butyrum,
"butter") is found in rancid butter, and caproic,
caprylic, and capric acids (Latin caper,
"goat") are all obtained from goat fat. A list of
common carboxylic acids is given in the table below.
Common
Carboxylic Acids
|
|
Common Name |
|
Formula |
|
Solubility in H2O
(g/100 mL) |
Saturated carboxylic acids
and fatty acids |
|
|
|
|
|
|
Formic acid |
|
HCO2H |
|
|
 |
|
|
|
|
|
|
|
|
Acetic acid |
|
CH3CO2H |
|
|
 |
|
|
|
|
|
|
|
|
Proprionic acid |
|
CH3CH2CO2H |
|
|
 |
|
|
|
|
|
|
|
|
Butyric acid |
|
CH3(CH2)2CO2H |
|
|
 |
|
|
|
|
|
|
|
|
Caproic acid |
|
CH3(CH2)4CO2H
|
|
0.968 |
|
|
Caprylic acid |
|
CH3(CH2)6CO2H
|
|
0.068 |
|
|
Capric acid |
|
CH3(CH2)8CO2H
|
|
0.015 |
|
|
Lauric acid |
|
CH3(CH2)10CO2H
|
|
0.0055 |
|
|
Myristic acid |
|
CH3(CH2)12CO2H
|
|
0.0020 |
|
|
Palmitic acid |
|
CH3(CH2)14CO2H
|
|
0.00072 |
|
|
Stearic acid |
|
CH3(CH2)16CO2H
|
|
0.00029 |
Unsaturated fatty acids |
|
|
|
|
|
|
Palmitoleic acid |
|
CH3(CH2)5CH=CH(CH2)7CO2H
|
|
|
Oleic acid |
|
CH3(CH2)7CH=CH(CH2)7CO2H
|
|
|
Linoleic acid |
|
CH3(CH2)4CH=CHCH2CH=CH(CH2)7CO2H |
|
|
Linolenic acid |
|
CH3CH2CH=CHCH2CH=CHCH2CH=CH(CH2)7CO2H
|
Naming
Carboxylic Acids
The systematic nomenclature of carboxylic acids is easy to
understand. The ending -oic acid is added to the
name of the parent alkane to indicate the presence of the
CO2H
functional group.
HCO2H |
|
|
Methanoic acid |
CH3CO2H |
|
|
Ethanoic acid |
CH3CH2CO2H |
|
|
Propanoic acid |
Unfortunately, because of the long history
of their importance in biology and biochemistry, you are more
likely to encounter these compounds by their common names.
Formic acid and acetic acid have a sharp, pungent odor. As
the length of the alkyl chain increases, the odor of
carboxylic acids becomes more unpleasant. Butyric acid, for
example, is found in sweat, and the odor of rancid meat is
due to carboxylic acids released as the meat spoils.
The solubility data in the table
above show that carboxylic acids also become less soluble in
water as the length of the alkyl chain increases. The
CO2H
end of this molecule is polar and therefore soluble in water.
As the alkyl chain gets longer, the molecule becomes more
nonpolar and less soluble in water.
Dicarboxylic
and Tricarboxylic Acids
Compounds that contain two
CO2H functional
groups are known as dicarboxylic acids. A
number of dicarboxylic acids (see table below) can be
isolated from natural sources. Tartaric acid, for example, is
a by-product of the fermentation of wine, and succinic,
fumaric, malic, and oxaloacetic acid are intermediates in the
metabolic pathway used to oxidize sugars to CO2
and H2O.
Common Dicarboxylic Acids
 |
|
oxalic acid |
 |
|
malonic acid |
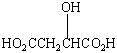 |
|
malic acid |
 |
|
succinic acid |
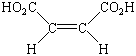 |
|
maleic acid |
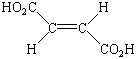 |
|
fumaric acid |
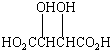 |
|
tartaric acid |
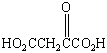 |
|
oxaloacetic acid |
Several tricarboxylic acids also play an
important role in the metabolism of sugar. The most important
example of this class of compounds is the citric acid that
gives so many fruit juices their characteristic acidity.

Esters
Carboxylic acids (-CO2H) can react with
alcohols (ROH) in the presence of either acid or base to form
esters (-CO2R). Acetic acid, for
example, reacts with ethanol to form ethyl acetate and water.
This isn't an efficient way of preparing an ester,
however, because the equilibrium constant for this reaction
is relatively small (Kc
3).
Chemists tend to synthesize esters in a two-step process.
They start by reacting the acid with a chlorinating agent
such as thionyl chloride (SOCl2) to form the
corresponding acyl chloride.
They then react the acyl chloride with an alcohol in the
presence of base to form the ester.
The base absorbs the HCl given off in this reaction,
thereby driving it to completion.
As might be expected, esters are named as if they were
derivatives of a carboxylic acid and an alcohol. The ending -ate
or -oate is added to the name of the parent
carboxylic acid, and the alcohol is identified using the
"alkyl alcohol" convention. The following ester,
for example, can be named as a derivative of acetic acid (CH3CO2H)
and ethyl alcohol (CH3CH2OH).
Or it can be named as a derivative of ethanoic acid (CH3CO2H)
and ethyl alcohol (CH3CH2OH).
The term ester is commonly used to describe the
product of the reaction of any strong acid with an alcohol.
Sulfuric acid, for example, reacts with methanol to form a
diester known as dimethyl sulfate.
Phosphoric acid reacts with alcohols to form triesters
such as triethyl phosphate.
Compounds that contain the
CO2R functional
group might therefore best be called carboxylic acid
esters, to indicate the acid from which they are
formed.
Carboxylic acid esters with low molecular weights are
colorless, volatile liquids that often have a pleasant odor.
They are important components of both natural and synthetic
flavors (see figure below).
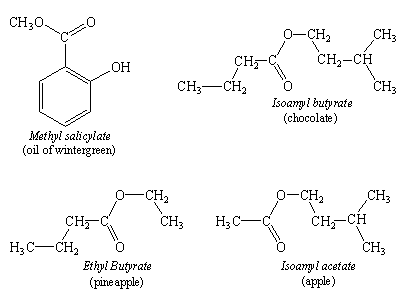

Fats and Oils
Long-chain carboxylic acids such as stearic acid [CH3(CH2)16CO2H]
are called fatty acids because they can be
isolated from animal fats. These fatty acids are subdivided
into the two categories on the basis of whether they contain
C=C double bonds: saturated fatty acids and unsaturated
fatty acids.
There are four important unsaturated fatty acids. One of
them is a derivative of palmitic acid, and is known as
palmitoleic acid.
The other three are derivatives of stearic acid. Oleic
acid has a single C=C double bond in the center of the fatty
acid chain.
Linoleic acid, has another C=C double bond in the nonpolar
half of the fatty acid chain.
Linolenic acid has one more C=C double bond in the same
half of the fatty acid chain.

There are several regularities in the chemistry of these
unsaturated fatty acids. First, they contain cis double
bonds. Second, the double bonds are always isolated from each
other by a CH2 group.
So much attention is paid to the structures of the fatty
acids in discussions of these compounds that it is easy to
miss an important point: Free fatty acids are seldom found in
nature. They are usually tied up with alcohols to form esters
(RCO2R). The most abundant of these esters is the
triester formed when a molecule of glycerol (HOCH2CHOHCH2OH)
combines with three fatty acids, as shown in the figure
below. These lipids have been known by a variety of names,
including fat, neutral fat, glyceride,
triglyceride, and triacylglycerol.
Most animal fats are complex mixtures of different
triglycerides. As the percentage of unsaturated fatty acids
increases, the melting point of these triesters decreases
until they eventually become an oil at room temperature. Beef
fat, which is roughly one-third unsaturated fatty acids, is a
solid. Olive oil, which is roughly 80% unsaturated, is a
liquid.
The effect of unsaturated fatty acids on the melting point
of a triglyceride can be understood by recognizing that the
cis C=C double bond introduces a rigid 30 bend in the
hydrocarbon chain. This bend or "kink" increases
the average distance between triglyceride molecules, which
decreases the van der Waals interactions between neighboring
molecules. Thus, the introduction of unsaturated fatty acids
into a triglyceride increases the fluidity of the lipid. The
table below compares the relative abundance of the common
fatty acids in a typical animal fat (butter) and a vegetable
oil (olive oil).
Relative Abundance of Fatty Acids in a
Typical Fat and a Typical Oil
Fatty Acid |
|
Butter |
|
Olive Oil |
Butyric |
|
3-4% |
|
|
Caproic |
|
1-2% |
|
|
Caprylic |
|
<1% |
|
|
Capric |
|
2-3% |
|
|
Lauric |
|
2-5% |
|
|
Myristic |
|
8-15% |
|
<1% |
Palmitic |
|
25-29% |
|
5-15% |
Stearic |
|
9-12% |
|
1-4% |
Palmitoleic |
|
4-6% |
|
|
Oleic |
|
18-33% |
|
67-84% |
Linoleic |
|
2-4% |
|
8-12% |
Linolenic |
|
<1% |
|
|
Fats and oils are used by living cells for
only one purpose
to store energy. They are a far more
efficient storage system than carbohydrates such as glycogen
or starch because they give off between two and three times
as much energy when they are burned. (Glycogen releases 15.7
kJ per gram of carbohydrate consumed, whereas lipids give
approximately 40 kJ/g.) This explains why the seeds of many
plants are relatively rich in oils, which provide the energy
the seed needs to grow until the leaves can begin to produce
energy by photosynthesis.
The average human contains enough fat (21% of the body
weight for men, 26% for women) to provide the energy they
need to survive for up to 3 months. But there is only enough
glycogen stored in the human body at any time to provide
enough energy for one day. Thus, glycogen is only used for
the short-term storage of food energy. In "times of
plenty," the body stores energy in the form of fat to
compensate for "times of shortage" to come.

